With the push towards environmentalism over the last few decades, there has been an explosion in the demand for new and innovative technologies to replace the monolithic fossil fuel industry. In this article we will explore issues with renewable energy sources, and how we can go about resolving them.
An important note about energy production is that, unlike the production of physical products, electricity is produced to directly match the consumption requirements predicted by a load forecast1. There is often very little, if any, storage along the way. This is because storing the massive amount of energy required for any given industrialized area using a conventional electricity storage medium such as lithium-ion batteries would amount to an unfathomable cost in implementation and repair*, and inertial means have been out of the question since the Gyrobus. Currently, we use power plants called “peaking plants” to compensate for sharp load differences during peak hours. However, these power plants are less efficient2 and more costly to run as a consequence of prioritizing rapid response over constant production.
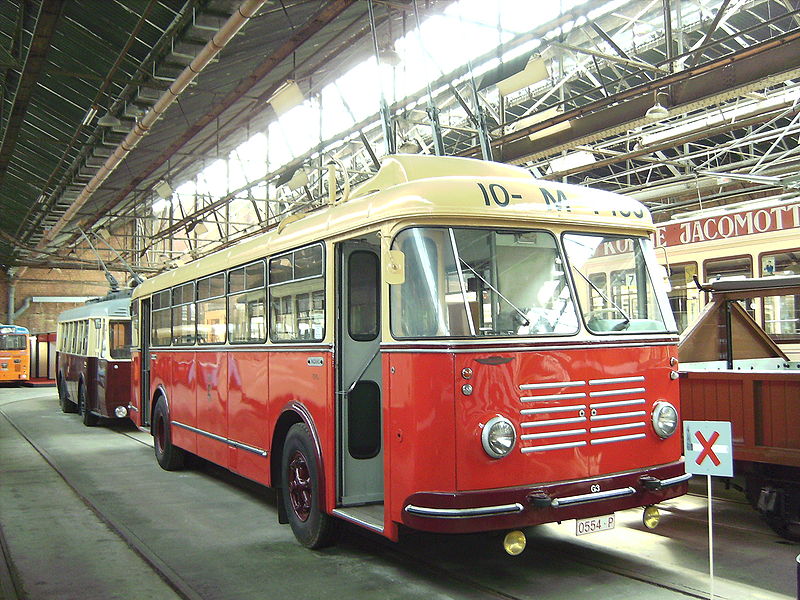
The Gyrobus was a mechanically interesting passenger vehicle driven by an internal flywheel. It was built in none other than the land of intricate mechanisms, Switzerland. The bus was charged by an electric motor when docked, however it was very inefficient and impractical overall. Image credit: Vitaly Volkov, Волков Виталий Сергеевич (user kneiphof) via Wikimedia Commons
One of the largest advantages that fossil fuels (and nuclear) have over renewable energy sources such as solar, wind and hydro, is that the output is predictable, consistent and controllable. Unsurprising, seeing as we can precisely regulate the fuel input. Conversely, when the air is stale or the sky is cloudy, the reliability of renewable sources is questionable at best. Combine this with a daily usage pattern that fluctuates on an hourly basis3, matching the populations’ load requirement with the necessary tolerances suddenly becomes a big problem without a significant buffer.
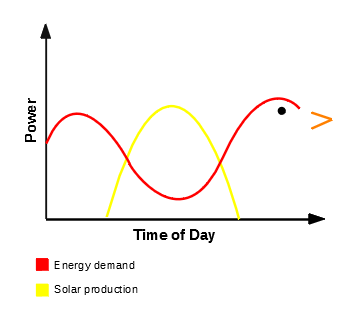
Quack! The “duck curve” represented here refers to the difference between solar production and demand for power. Solar production peaks at midday, however generally our demand peaks in the mornings and evenings.
With the advent of the reversible hydroelectric turbine-pump in the 1930s, water has been used effectively as a means of storage by pumping it up into a reservoir when energy production exceeds consumption and releasing it when demand exceeds supply. In yet another nod to the engineering marvels of Switzerland, they were the first to use this form of energy storage in 1907.
This method is feasible in particular due to its comparative scalability. Water is cheap – it doesn’t just grow on trees; trees grow on it. This compensates for the fact that the energy density of a mass of water at height is dwarfed by other means such as gasoline or batteries. The largest cost associated with this technology is the construction of dams, which are always damn large civil engineering escapades.
Another advantage of using pumped storage dams is that the turnaround is very fast. Open the valves and a dam can begin producing megawatts almost instantaneously and controlled, and closing the valves is just as effective. This provides a replacement for the inefficient peaking stations when the demand slope is great.
In a world that is constantly developing, industrializing, and one-upping last year’s consumption of everything (especially toilet paper), increasing our use of this technology could help us sate our energy needs by making solar and wind more versatile. When accompanied by the constant output of nuclear, we could one day phase out fossil fuels for good.
* Ontario consumes roughly 16,000 MW at midnight (lowest consumption period) at the time of writing4. A mere hour of this amounts to a staggering 57 terajoules (5.7×10^13 J), and to store that with lithium-ion batteries (maximum energy density of 0.875 MJ/kg5) would require a battery that weighs 65,000 metric tons, or about 1,140 M1 Abrams Main Battle Tanks. At an approximate cost of CA$170.07 (US$125) per kg6, this much battery mass would cost, very conservatively, CA$1.1Bn.
FURTHER LEARNING:
Practical Engineering – https://www.youtube.com/watch?v=66YRCjkxIcg
VOX – https://www.youtube.com/watch?v=YYLzss58CLs
- https://www.energy.gov/sites/prod/files/2017/01/f34/Maintaining%20Reliability%20in%20the%20Modern%20Power%20System.pdf (p.7)↩
- https://www.sciencepolicycircle.org/38-the-peakers-the-role-of-peaking-power-plants-and-their-relevance-today↩
- https://www.eia.gov/todayinenergy/detail.php?id=830↩
- http://www.ieso.ca/Power-Data/This-Hours-Data↩
- https://www.cei.washington.edu/education/science-of-solar/battery-technology/↩
- https://qz.com/1588236/how-we-get-to-the-next-big-battery-breakthrough/↩
Leave a Reply